Research
Current Research Directions
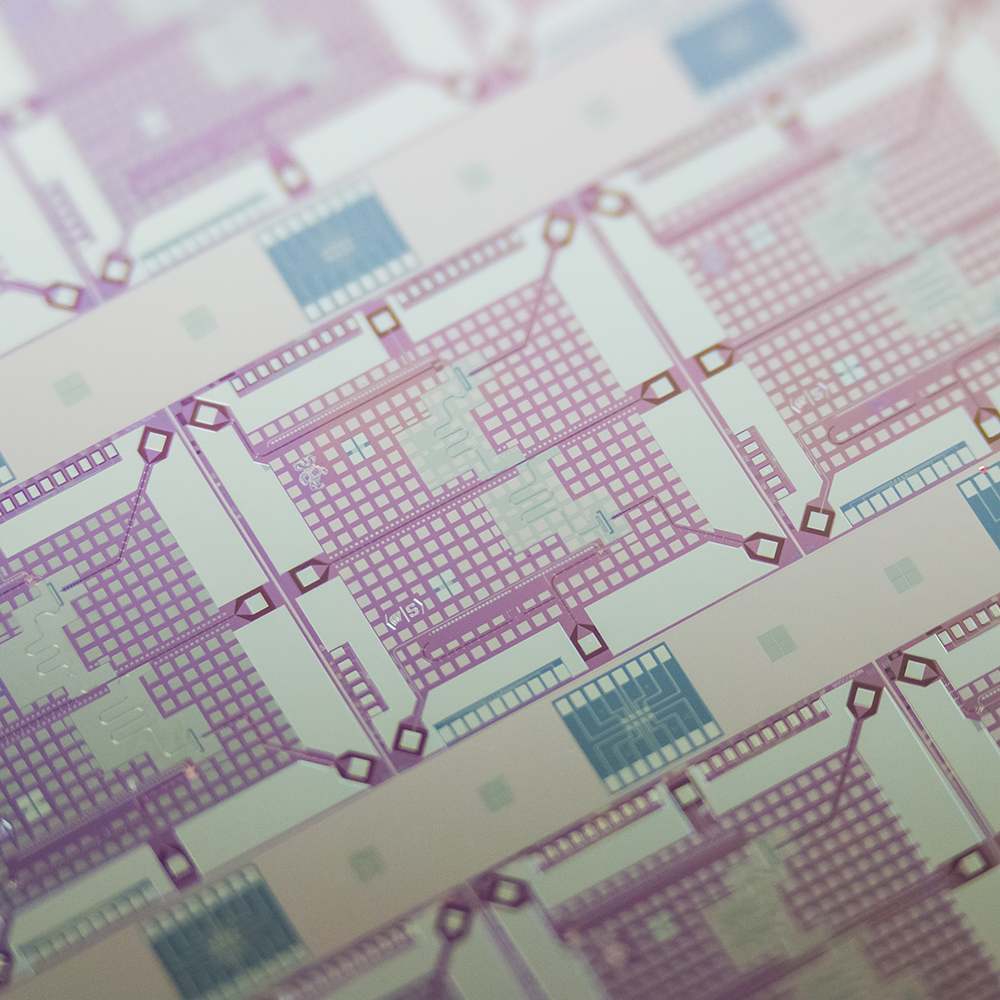
Quantum Coherence
State preservation is crucial for any application of quantum technology. Noise and dissipation lead to the irretrievable loss of quantum information. In frequency-tunable qubits, low-frequency magnetic flux noise is a dominant source of qubit dephasing. Similarly, surface dielectric two-level state (TLS) defects lead to enhanced qubit energy relaxation. In our lab, we are working to understand the microscopic origins of these noise sources and developing methods to mitigate their effects. Collaboration with L. Faoro, L. B. Ioffe
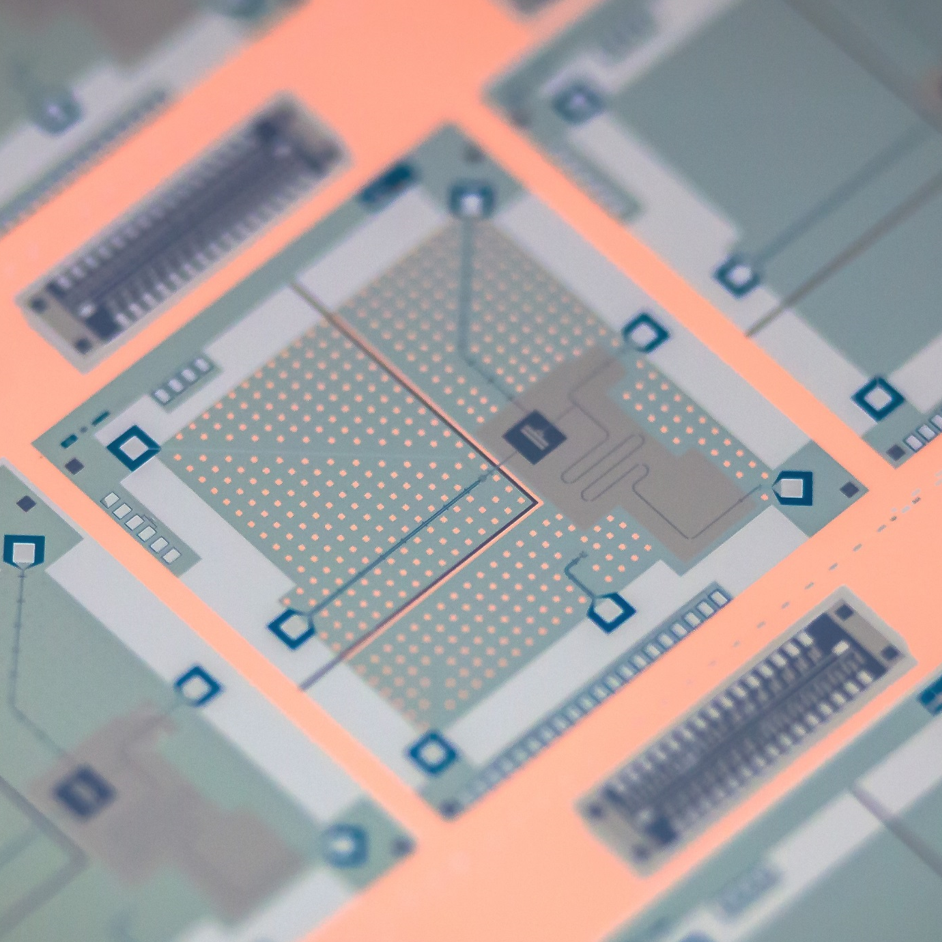
Scalable Coherent Control
Fault tolerance demands high-fidelity control of the qubit state. Conventionally, coherent rotations are achieved via shaped microwave tones that realize arbitrary rotations over the Bloch sphere; however, the room temperature electronics overhead and latency associated with this approach preclude scaling to large quantum arrays. We have developed an alternate control scheme involving irradiation of the qubit with trains of quantized flux pulses derived from the Single Flux Quantum (SFQ) digital logic family. This approach opens the door to realization of a scalable classical coprocessor integrated at the millikelvin stage for low-latency feedback and stabilization of the quantum array. Collaboration with B. L. T. Plourde, M. G. Vavilov, F. K. Wilhelm
Quantum Measurement
Quantum error correction requires fast, high-fidelity qubit measurement. We are pursuing two approaches to qubit measurement, one based on linear amplification using a variant of the dc Superconducting QUantum Interference Device (dc SQUID) and one based on entanglement of the qubit with a linear cavity followed by photon counting. In both cases, emphasis is on scaling to large qubit arrays and elimination of nonreciprocal components in the measurement chain. Collaboration with B. L. T. Plourde, M. G. Vavilov, F. K. Wilhelm
Hybrid Quantum Systems
We are pursuing development of hybrid quantum systems that will capitalize on the unique strengths of disparate quantum technologies. One goal is realization of an optimized hybrid quantum computer that will combine a high-speed superconducting quantum processor with a long-lived atomic quantum memory. We have developed a protocol for high-fidelity superconductor—atom entanglement and we have constructed a 4 Kelvin testbed for preliminary experiments to couple a high-Q niobium cavity waveguide with a single trapped Rydberg atom. In other work, we are adapting concepts from superconducting circuit quantum electrodynamics to the high-fidelity readout and control of semiconducting qubits. Collaboration with M. Saffman and M. A. Eriksson
Facilities
Measurement
The McDermott group has commissioned five low-temperature measurement platforms:
- Three adiabatic demagnetization refrigerators with base temperature around 50 mK. These systems are used for dc and microwave transport measurements and investigations of excess 1/f noise in Josephson devices.
- Two large-wire count dilution refrigerators with base temperature around 20 mK. These systems are dedicated to Josephson qubit measurements.
Device Fabrication
Our laboratory has two sputter deposition systems and one electron-beam evaporator for the growth of high-quality superconducting thin films and tunnel junctions.
The Wisconsin Center for Applied Microelectronics (WCAM) offers state-of-the-art facilities for thin film deposition and reactive ion etching, along with advanced UV projection lithography and electron beam lithography.